
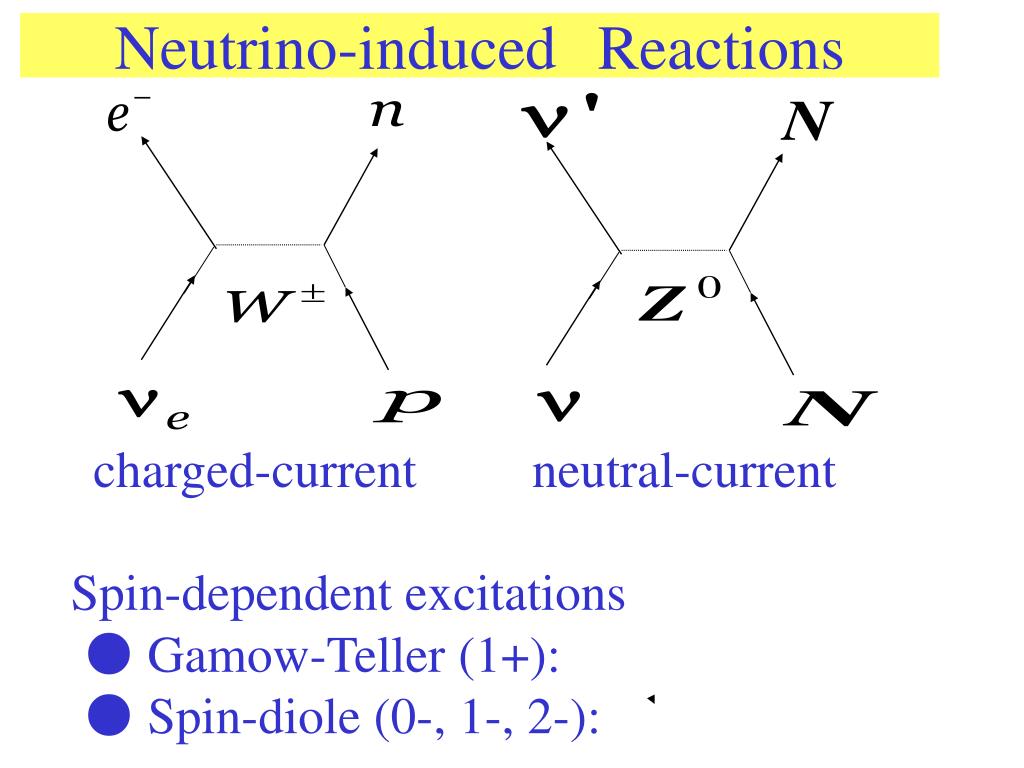
Janka HT (1993) Neutrinos from type-II supernovae and the neutrino-driven supernova mechanism. Horowitz CJ (2002) Weak magnetism for antineutrinos in supernovae. Hirata K, Kajita T, Koshiba M, Nakahata M, Oyama Y (1987) Observation of a neutrino burst from the supernova SN 1987A. Hannestad S, Janka HT, Raffelt GG, Sigl G (2000) Electron-, mu-, and tau-number conservation in a supernova core. doi: 10.1086/148549įreedman DZ, Schramm DN, Tubbs DL (1977) The weak neutral current and its effects in stellar collapse. astro-ph/0404432Ĭolgate SA, White RH (1966) The hydrodynamic behavior of supernovae explosions. astro-ph/9905132īurrows A, Reddy S, Thompson TA (2006) Neutrino opacities in nuclear matter. doi: 10.1086/164405īurrows A, Young T, Pinto P, Eastman R, Thompson TA (2000) A new algorithm for supernova neutrino transport and some applications.
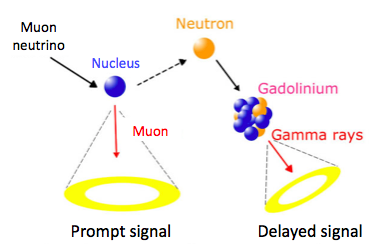
Springer, New York, pp 143–181īurrows A, Lattimer JM (1986) The birth of neutron stars. astro-ph/0507135īurrows A (1984) On detecting stellar collapse with neutrinos. Numerical method and results for a 15 M ⊙ star. doi: 10.1086/191056īuras R, Rampp M, Janka HT, Kifonidis K (2006) Two-dimensional hydrodynamic core-collapse supernova simulations with spectral neutrino transport. doi: 10.1103/PhysRevLett.58.1494īruenn SW (1985) Stellar core collapse – numerical model and infall epoch. doi: 10.1103/RevModPhys.62.801īionta RM, Blewitt G, Bratton CB, Casper D, Ciocio A (1987) Observation of a neutrino burst in coincidence with supernova 1987A in the Large Magellanic Cloud. Can J Phys 44:2553īalasi KG, Langanke K, Martínez-Pinedo G (2015) Neutrino-nucleus reactions and their role for supernova dynamics and nucleosynthesis. astro-ph/0612582Īrnett WD (1966) Gravitational collapse and weak interactions. Spherically symmetric hydrodynamic simulations. doi: 10.1016/0370-2693(88)91651-6Īrcones A, Janka HT, Scheck L (2007) Nucleosynthesis-relevant conditions in neutrino-driven supernova outflows. This includes the most relevant neutrino production, absorption, and scattering processes, elementary aspects of neutrino transport in dense environments, the characteristic neutrino-emission phases with their typical signal features, and the perspectives connected to a measurement of the neutrino signal from a future galactic supernova.Īlexeyev EN, Alexeyeva LN, Krivosheina IV, Volchenko VI (1988) Detection of the neutrino signal from SN 1987A in the LMC using the INR Baksan underground scintillation telescope. In this chapter the basic neutrino physics in supernova cores and nascent neutron stars will be discussed. The absorption of electron neutrinos and antineutrinos in the surroundings of the newly formed neutron star can power the supernova explosion and determines the conditions in the innermost supernova ejecta, making them an interesting site for the nucleosynthesis of iron-group elements and trans-iron nuclei. The emission of neutrinos and antineutrinos of all flavors carries away the gravitational binding energy of the compact remnant and drives its evolution from the hot initial to the cold final state. The release of electron neutrinos, which are abundantly produced by electron captures, accelerates the catastrophic infall and causes a gradual neutronization of the stellar plasma by converting protons to neutrons as dominant constituents of neutron star matter. These elementary particles play a crucial role when the evolution of a massive star is terminated by the collapse of its core to a neutron star or a black hole and the star explodes as supernova. Supernovae are the most powerful cosmic sources of MeV neutrinos.
